|
|
|
Articles > Hight Transparency Structures |
|
|
|
|
|
|
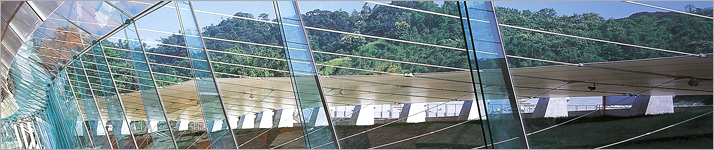 |
|
Hight Transparency Structures |
|
Mic Patterson
|
Principal,CEO
ASI(Advanced Structures Incorporated is an international consulting and design/build firm)
|
The vision is a spiders web of structure with a soap bubble film. Intricate Minimalist filigree structures floating in a sea of light. Seamless glass membranes spanning vast spaces, disappearing with a shimmer, reappearing in a faint reflection of their surroundings. Such has been the stuff of dreams for building designers since the mid 19th Century when the construction of the great iron and glass conservatories in Europe and England first demonstrated the exciting potential of glass in architecture. Today these dreams are being realized repeatedly in the construction of increasingly innovative glass structure. The overriding objective of this new genre of structure is maximized transparency, and its most common form is the long span structure glass façade, although roof structures and complete building enclosures have been realized. What are the issues with respect to these High Transparency structures; what are the methods, tools and techniques at the designers disposal in their realization? |
|
Glass as Material
Glass is a material of adventure and exploration, constantly evolving, expanding in its application and improving in its performance. Glass is the most exciting and dynamic material available to the building designer today. Very few materials demonstrate such range and flexibility in architectural application. Compared to metallic or masonry materials, glass material science is a relative hotbed of material delopment and innovation. New glass processing techniques, laminating materials, coatings and films have greatly widened the performance spectrum of glass in a number of measures. These developments, along with increasingly sophisticated engineering know-how, have vastly widened the application of glass as material, from building structure to building skin.
Beyond Windows
Developments with respect to the toughening of glass and its fabrication into layered and composite assemblies to improve structural properties, have allowed for the evolution of glass beyond a mere cladding element and provided for its application as a full fledged structural material. Glass has now been successfully employed as virtually every conceivable building component: from windows and doors to posts and beams, as stair treads and leadings, as floors and ceilings and walls, as complete building enclosures.
|
|
uconn蛛網結構-不銹鋼珠網結構支撐穿孔點支撐隔熱玻璃板。
|
|
|
|
|
|
.jpg)
|
|
HA-LO大樓高穿透性立面與色板玻璃 - 美國伊利諾州由 Murphy / Jahn Architects 設計之HA-LO大樓,入口與立面上層的牆與屋頂運用低含鐵量玻璃,與其他部位運用的藍色玻璃有明顯之區別。
|
Point-fixed Glazing Systems
The evolution of high transparency structures is firmly linked to the development of point-fixed glazing systems. Basically these systems involve techniques to mechanically fix the glass to a backer structure.
One class of system requires that the glass panel be drilled to receive a fitting that provides for the bolting of the glass panel to the backer structure. Variations include through-bolting, through-bolting with no penetration of the outer glass surface (laminated and insulated panels only). Fittings include clamp and articulated versions, with differences in how they load the glass.
Another class of system eliminates the need for drilling holes in the glass, and involves a “patch”or “pinch”component that effectively clamps the glass to the backer structure. While this system saves the cost of glass drilling, it typically results in an exterior plate on the glass grid.
A great benefit of these systems is that they allow for the glass membrane to be stepped away from the backer structure, often via the use of a stainless steel component referred to as a spider, which picks up four adjacent corners of glass at a vertex of the glass grid. This visually lightens the structure and enhances transparency.
Transparency in Architecture
The single most compelling attribute of glass relates to its properties of transmittance, particularly with respect to the visible light spectrum. In its natural state, glass has the extraordinary property of transparency. It is this property that allowed the opening up of dwellings and buildings to daylight and view, while simultaneously providing some level of protection against natural elements.
Transparency has been a prominent feature of architecture since the early 20th century, when new technology simplified the glass manufacturing process providing more and better material at less cost. Technology again influenced the use of glass in architecture with the introduction of the float glass process developed by Alastair Pilkington in the late 1950s.
The evolution of transparency in architecture continues unabated. The building designer today is armed with powerfull new materials, tools and information that have provided for the construction of an exciting new body of high transparency structure.
|
.jpg) |
|
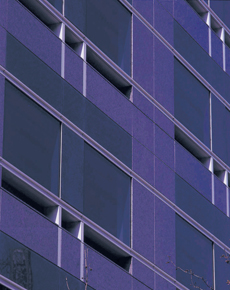
|
上海新威斯汀飯店中庭屋頂John Portman & Associates 所設計之上海新威斯汀飯店,施予張力之不銹鋼索桁架作為點支撐無鑽孔隔熱平板玻璃之構造基礎。 |
|
Kevin Roche John Dinkloo and Associates 作品 紐約大學Kimmel 中心:特殊訂製之懸吊張力網提供精彩之視覺效果。 |
|
Techniques of Transparency
The basic raw material of glass is sand. Iron oxide, present as a trace impurity in the sand, gives glass its greenish tint. It also effectively reduces the transmittance of the glass. Manufacturing processes have been developed to reduce these trace impurities, resulting in a “low-iron”glass product, also referred to variously as super-clear or clear-white glass. The reduction of the iron oxide in the raw material effectively removes the greenish tint and increases the transmittance properties of the glass. The increased transparency of low-iron glass is readily apparent.
Another significant attribute of glass is its property of reflectance. Glass reflects light as a function of the material makeup, surface coating, and the angle of incidence of the light (the greater the angle, the higher the reflection). Surface coatings such as the so called mirror coatings dramatically increase reflection properties. However, even normal glass reflects light. In fact, it is primarily through reflection, the reflected images on a glass surface, that we see glass. Take away the reflections, the glass “disappears”and transparency is enhanced. One of the many types of interference coating available for glass addresses just this, the reduction of reflected light. Anti-reflective coatings significantly reduce reflected light from the glass surface, particularly at angles of incidence near normal to the glass plane. The effect is to dramatically increase the perception of transparency of the glass, especially when viewed straight on.
Transparency and Tension
The effort to dematerialize the structural component in high transparency facades leads inexorably to the employment or structural designs maximizing the utilization of tensile elements. If a structure can be designed such at certain structural components can be isolated in a load transfer system where they will only be subject to tension forces, such members can be significantly reduced in size. For example, a 100mm tubular compression member can often be reduced to a cable or rod tension member of 10mm diameter or less. Depending on loading conditions, 20mm cables properly pre-tensioned and as part of a cable net structural system, can accommodate a glass façade spaning over 30 meters.
This phenomenon has given rise to the extensive development and use of sophisticated tension structures in support of high transparency, long span facades. Tensioned cable trusses have been used in a great variety of configurations to support point-fixed glazing systems. Their application has ranged from long span glass walls and roofs, to highly custom designs with compression members suspended in a network of cables or rods.
The cable truss roof pictured here encloses the lobby of the new Westin Hotel in Shanghai, designed by John Portman & Associates, Inc of Atlanta, Georgia. The glass roof spans 30 meters. Insulated and laminated glass panels are point-fixed without any requirement for drilling via an innovative clamping technique utilizing a thin blade that penetrates through the glass seam.
Most recently, the push for transparency has resulted in the employment of cable net structures for the support of large span facades. In its most basic form, this structure type is characterized by a flat net created from steel (most often stainless steel) cables tensioned vertically and fixed at all vertices with a specially designed component that both clamps the cables to prevent any slippage and provides for the fixing of the glass to the vertex component. The glass fixing in this case most often takes the form of a corner clamp; the vertex component incorporates a shelf on which the glass corner sit, and a final exterior cap bolts to the vertex component, effectively clamping the glass to the net. Thus, any glass makeup is easily accommodated, and the expense of drilled glass is avoided. A simple butt-glazed silicone seal is fried applied to provide the final weather barrier.
Blurring the Boundaries
In 1945 John Entenza or Arts and Architecture magazine initiated the Case Study program in which prominent architects of the time developed experimental prototypes of some 36 houses, the majority of which were built over the following years in the Southern California area. The Case Study House program had an enormous influence on architects and the course of architecture itself. Many of these houses featured and ultimately popularized the incorporation of large areas of floor to ceiling glass in an effort to mitigate the perceived boundary between inside and outside. This was precisely the stated intent of Lohan Caprile Goettsch Architects in Chicago for the street level lobby area of a new downtown high-rise. A cable net strategy was selected to provide the most minimum structural profile, and a low-iron glass with an anti-reflection coating was specified for the glass material. The glass was used as a fully tempered monolithic sheet.
The result is a lobby space with only the most minimal, highly transparent membrane, like a soap bubble film, separating it from the outside pedestrian environment. When viewed normal to the glazed surface, the reflection free glass virtually disappears. In addition to boasts perhaps the most highly transparent glass façade yet constructed, as a function of the qualities of the glass utilized in its construction.
Flat cable net facades, having no depth and relying solely on pre-tensioning to resist dynamic loading, are subject to considerable deflection. The most common deflection design criteria for this façade type is L/50, meaning at flat cable net façade spaning 30 meters will be subject to maximum deflection under wind load of .6 meters. The other predominant design issue relative to such facades is the relatively high magnitude of force transferred to the supporting boundary structure by the cable net. The boundary structure must be engineered to withstand the high cable pre-tension forces, and any additional forces the façade will induce under maximum design load. This typically necessitates the installation of heavy structure at the boundary interface between the cable net façade and the building structure.
|
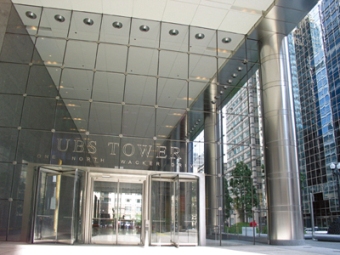
|
|
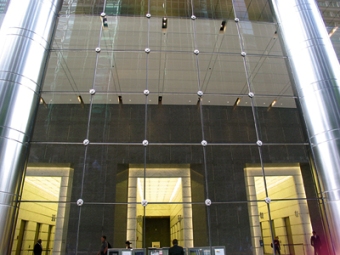
|
UBS大樓入口:芝加哥 UBS 大樓由 Lohan Caprile Goettsch 事務所設計,(左圖)入口由地面懸臂與襯墊支撐以抵抗鋼索與入口間之力傳導;(右圖)防反射之塗覆使正向觀看玻璃達到完全之穿透感。 |
|
|
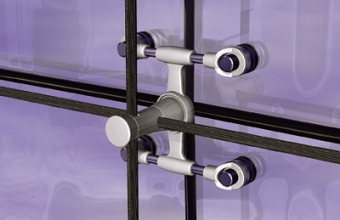
Fentress Bradburn Architects LTD 之作品-西雅圖西塔國際機場,雙弧面玻璃帷幕作為穩定的玻璃結構減少鋼索往構造之側向撓曲。
(上圖)穿孔之玻璃平板由不銹鋼構件予以點支撐抗動態荷重。
|
|
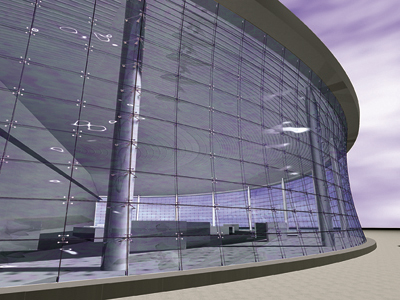
|
|
|
A New Twist
The most recent development with the cable net facades involves designing the cable net membrane as a double-curved surface as opposed to a simple flat grid. The opposing curvatures and resulting saddle shaped surface act to provide an inherent geometric stability lacking in the flat cable nets, exactly as what takes place with tensioned fabric membrane structures. A flat, square fabric awning, for example, will flap in the wind, such flapping moderated only in a limited fashion applying tension to the fabric. However, if one set of opposing corners is pulled in the other direction, a saddle shape will result and the membrane will be stable under significantly higher wind loading.
The same principal applies with the cable net. The primary advantage of this design strategy, in addition to providing a more interesting surface geometry to the façade, is that the enhanced stability of the double-curved net significantly reduces the high deflections associated with the flat nets, and thus requires less pre-tensioning to control those deflections. This reduces the requirement for a heavy supporting boundary structure to resist these loads.
A new façade design under construction at Sea-Tac (Seattle-Tacoma) Airport utilizes a double-curved surface geometry. This design takes on the additional complexity of incorporating drilled, point-fixed insulated glass panels. The geometry of the curved net results in a proliferation of non rectangular shapes. In addition, the geometry of this net results in some locations where the 4 vertices at certain grid locations do not lie on the same plane, necessitating the warping of the glass panels in the installed condition. Additional warping occurs under dynamic load. Each individual glass panel in the design was analyzed and engineered to withstand these warping forces in addition to other dynamic loads.
Structural Glass Components
Another technique to dematerialize the structure and enhance transparency in long span facades is to substitute glass components for conventional metallic components. The most common examples of this are glass fin supported facades, popularized by the glass wall for the Willis Faber & Dumas building designed by Foster Associates in 1972. A glass fin, monolithic or laminated, is positioned perpendicular to the glass plane at the vertical glass seam to stiffen the wall against wind loads. Early systems utilized a patch fitting to attach the glass, effectively restraining the glass at its corners while providing for building and thermal movement. Contemporary systems commonly use drilled glass panels and countersunk stainless steel fittings to fix the glass to the fin.
Recent structures have begun to incorporate glass beam elements in a more innovative manner. In the example pictured here, a laminated glass beam spans between truss elements to provide transparent support to the glazing grid.
|
|
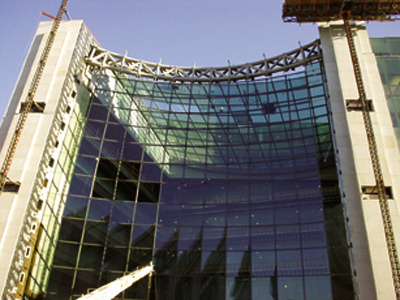 |
|
SEC 大樓立面:Kevin Roche John Dinkloo and Associates LLC 之作品-華盛頓特區 SEC 大樓。其雙弧面玻璃帷幕跨距20公尺高30公尺,天窗為20公尺見方。
|
Opportunities with Insulated Glass
Compromise is often called for in the pursuit for transparency. Energy concerns often mandate the use of insulated glass panels, which are inherently less transparent than a simple single sheet monolithic panel. In addition, the seam formed by the sealant joint between two insulated glass panels combines with the spacer separating the inner and outer glass in each panel, this total dimension comprising an opaque band around each glass panel in the final installation. The visual effect of this opaque band significantly impacts the visual aesthetic of a glass wall using insulated glass verses monolithic glass, rendering the insulated glass façade somewhat less transparent, independent of other considerations such as the supporting structure. Given the frequent necessity of insulated glass as a performance requirement, designers have discovered that they can add thin metallic framing elements to the glass panel without significant further compromise of transparency. The frame components are structurally glued to the back of the glass panel. The panel is then point fixed off the framing element and tied back to the supporting structure. In this condition conventional glass can be used, as the glass itself is not subject to point loading.
The great benefit here is that the glass plane can be lifted off the supporting structure as it is with most structural glass systems, significantly enhancing the apparent transparency of the glass structure. The premium cost associated with drilled and point-fixed glass s avoided. The result is transparency that rivals that of the most expensive structural glass facades at a significantly reduced cost. This framed or panelized strategy also facilitates field assembly over the drilled glass systems, further improving the economic efficiency of this system type.
An example of this approach is pictured here; a constructing shot of a glass façade and skylight for the Securities and Exchange Commission Corporate Headquarters in Washington, D.C. The lobby area is enclosed by a double-curved glass façade joined by a curved triangular truss to a double-curved skylight. Insulated glass is used in a prefabricated panelized system. Skylight glass assemblies are insulated and laminated as required by code for overhead glazing.
A Transparent Future (Sometimes)
High transparency will remain a common theme in architecture, with the progressive integration of glass and structure, and the continued development of glass and glass processing, making the realization of high transparency both easier and cheaper. Performance enhancements of energy performance as a barrier to high transparency, and provide higher strength products capable of replacing more metallic structural components.
However, high transparency is obviously not appropriate or desirable in many applications, at least not full time transparency, and here lies perhaps the most exciting opportunity; variable transmission facades capable of selective and varying transmittance, from highly transparent to opaque, in response to any number of internal or external conditions or direct occupant input. Time, temperature, and light level in any combination are few of the parameters by which a dynamic façade will be programmed to provide improved energy performance and comfort.
Building facades of the future will be dynamic skins integrated not only with the supporting structure, but with all building systems, thus playing a roll in everything from energy production and conservation, to natural and artificial lighting, ventilation, heating and cooling, and even communication. The developments that are in the works to make this possible will provide the creative building designer with the tools necessary to better combine the functional and aesthetic objectives of architecture. The new glass products resulting from this development will surely play a prominent role in future architecture.
|
|
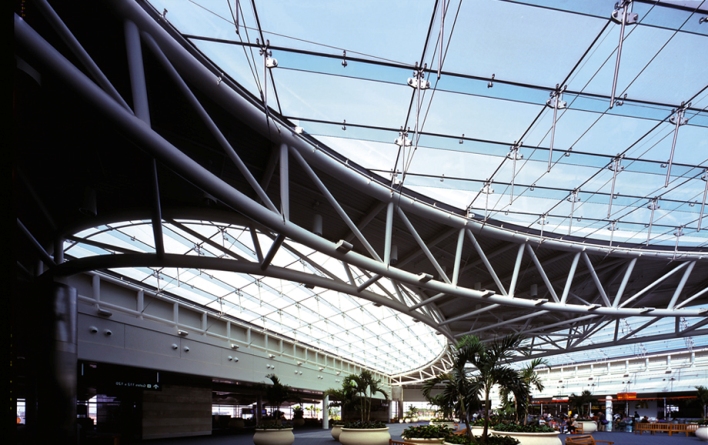 |
|
|
|
|